A recent experiment at Fermilab has observed a large asymmetry between the up and downstream ocean quark distributions of protons.
The first direct evidence of point-like components in nucleons comes from the observation of scaling phenomena in deep elastic scattering (DIS) experiments. The charged components, such as these points, known by Richard Feynman, are found to be spin-1/2 fermions. These partons were initially identified as valence Quarks in the component Quark model.
However, it was quickly recognized that only Valence Quarks could not explain the low momentum parton herd observed in DIS. These low-moment partons, known by Feynman, were interpreted as the quarks and anti-cruke seas of nucleon. Therefore, the DIS experiment provided the first evidence of the presence of bone charcoal in the nucleons.
Parton’s observation in the DIS experiment paved the way for the formulation of quantum chromodynamics (QCD) as a powerful theory of interaction.
Nevertheless, the exact form of the Parton distribution function (PDF) cannot be estimated from the perturbed QCD. Like many static properties of hadrons, PDFs belong to the domain of non-perturbed QCDs.
Despite significant advances in lattice gauge theory (LGT) in dealing with hadron bond state properties, predicting PDF using LGT remains a challenge.
Initial DIS data suggest that the nucleons consist of three quarks in the background of an infinite number of Quark-Antiquark pairs. The importance of the quark antikica pair in nucleon is in contrast to the situation in atomic systems where the particle antiparticle pair plays a relatively minor role.
In strong interactions, Quark-Antiquark pairs are easily generated as a result of the relatively large magnitude of the coupling constant α, forming an integral part of the nucleon structure.
There are at least two reasons why it is important to measure the parton distribution function of nucleons.
First, a parton distribution function is required as an essential input to explain hard processes in high energy interactions. Second, many aspects of the parton distribution can be compared with predictions of theoretical models based on perturbation and non-perturbed QCD.
We present a state of knowledge regarding the asymmetry of the flavor of the proton light quark sea. Opportunities for further investigation of this subject with existing accelerators and future electron ion corridors (EICs) will also be discussed.
Flavor Asymmetry from DIS
The oldest Parton model assumed that the Proton Sea was flavor symmetric, despite the clearly asymmetric valence Quark distribution. Inherent to this assumption is that the marine content is independent of the composition of the valence quark. Therefore, protons and neutrons were expected to have the same ocean distribution. The assumption of flavor symmetry was not based on known physics and remained tested by experiments.
Experiments using neutrino beams provided strong evidence that the nucleon’s strange quark content is only about half of the quarks in the ocean above or below. The asymmetry of this flavour was attributed to the very heavy mass of the strange quarks compared to the top and bottom quarks.
It is suggested that the masses of the upper and lower quarks are very similar. This suggests that the nucleon sea should be roughly up and down symmetrical. A direct way to confirm this assumption is to compare the neutron ocean with the proton ocean measured in the DIS experiment.
Shortly after the discovery of partons in electron proton DIS, electron scattering experiments were carried out to extract electron-neutron DIS. The EP-P and E-N DIS data allow comparison of the quark distributions in the up and down seas of protons. Despite the large uncertainty of the experimental data, Field and Feynman speculated from these data that the quarks in the upper and lower seas differed in the protons.
They further suggested that Paulies blocked from valence quarks produce asymmetric proton seas as they inhibit the Quark Sea more than the Quark Sea.
However, large-scale experimental uncertainty prevented a critical conclusion. A more accurate DIS experiment was later carried out by an NMC collaboration at CERN, indicating that the content of quarks in the up and down seas of proton differs at a confidence level of 4σ. The Drell-Yan process described below provides a more direct and definitive measure of this unexpected asymmetry in the proton sea.
Flavor Asymmetry from the Drell-Yan Process
The first high-mass dylepton production experiment was carried out at AGS in 1969 shortly after the DIS experiment was carried out at SLAC. Drell and Yan interpreted the data in the Parton model. In this model, the Quark-Antiquark pair is annihilated by virtual photons and then collapses into Lepton pairs (see Figure 1).
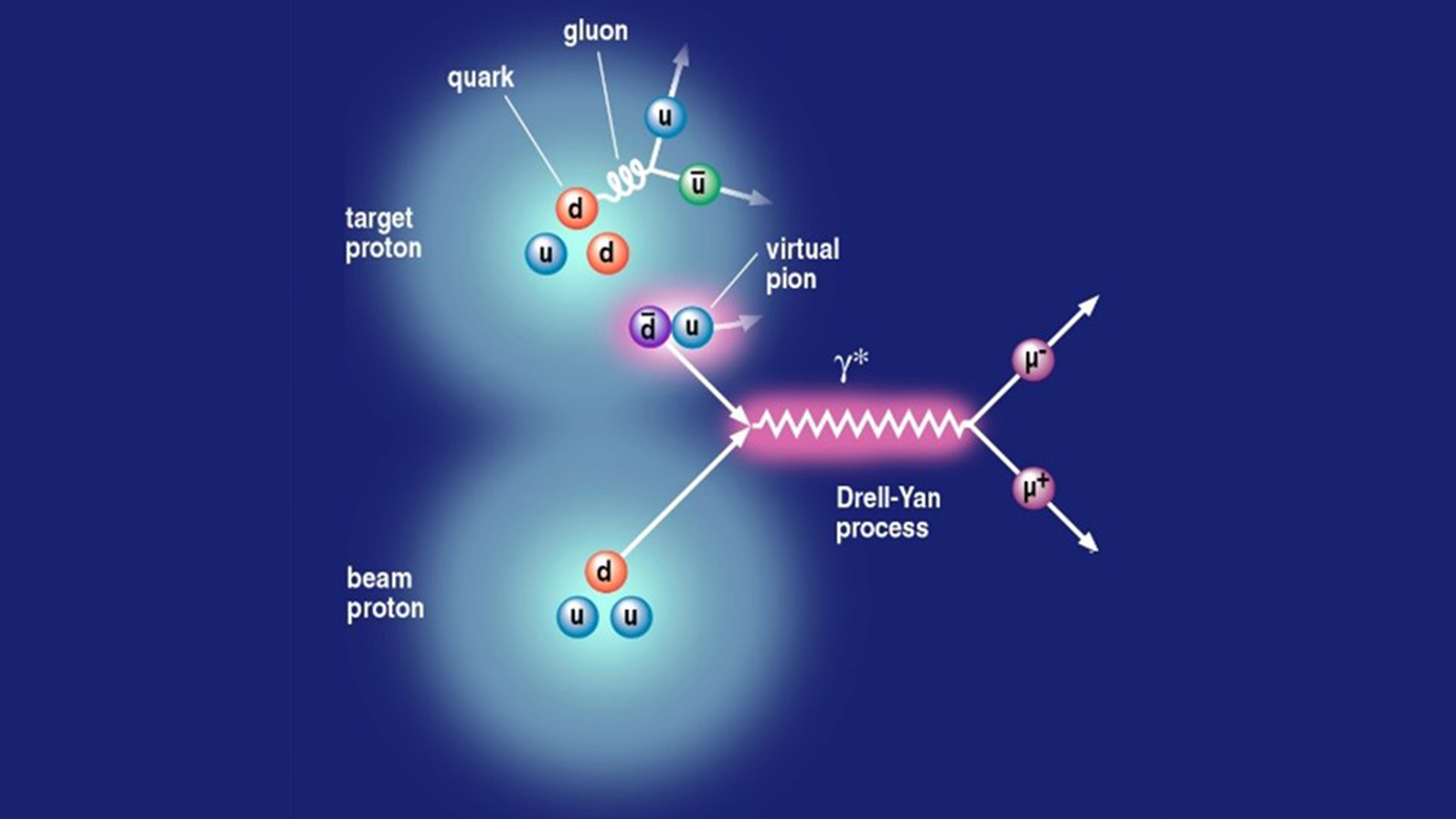
The target proton meson cloud is highlighted.
This simple model can explain some related features of the data. Therefore, the production of high-mass continuum lepton pairs is called the Drell-Yan (DY) process. The underlying mechanism of the DY process involves the disappearance of quarks in the antiques, making it ideal for investigating the antiques of beams or target hadrons.
Apart from the DIS experiments, an independent and elegant method for testing the asymmetry of the light quark sea flavour is the proton-induced DRELL-YAN process. The collaboration of Fermilab E772 was first compared to the generation of Dy Muon pairs from proton-rich targets with the non-quality constraints of proton U̅ and D̅.
Subsequently, CERN experiment Na51 performed yield comparisons of Dy Muon pair yields from hydrogen and deuterium and found that d̅ was greater than twice as high at x = 0.18. By Antikruk.
In Fermilab, higher statistics are much wider than the NA51 experiment, which collected 330,000 DY events using three different spectrometer events covering low, medium and high-mass moon regions. This is intended for DY experiments (E866/Nucea) with precision. pair. In the E866 experiment, we found that u̅ is in fact different from d̅, as shown in Figure 2. By covering a wide range of motion, the E866 experiment revealed an interesting x dependency on d̅(x)/u̅(x) asymmetry. . If the X value is lowest, u̅(x) and d̅(x) are equivalent. As x increases, d̅(x)/u̅(x) increases monotonically, reaching a maximum of about 1.75 at x≈0.15. At even higher X values, the E866 data show that d̅(x)/u̅(x) begins to drop, less than unity at the highest x values. The flavor asymmetry between u̅(x) and d̅(x) was also observed by Hermes’ collaboration using semi-comprehensive deep inelastic scattering.
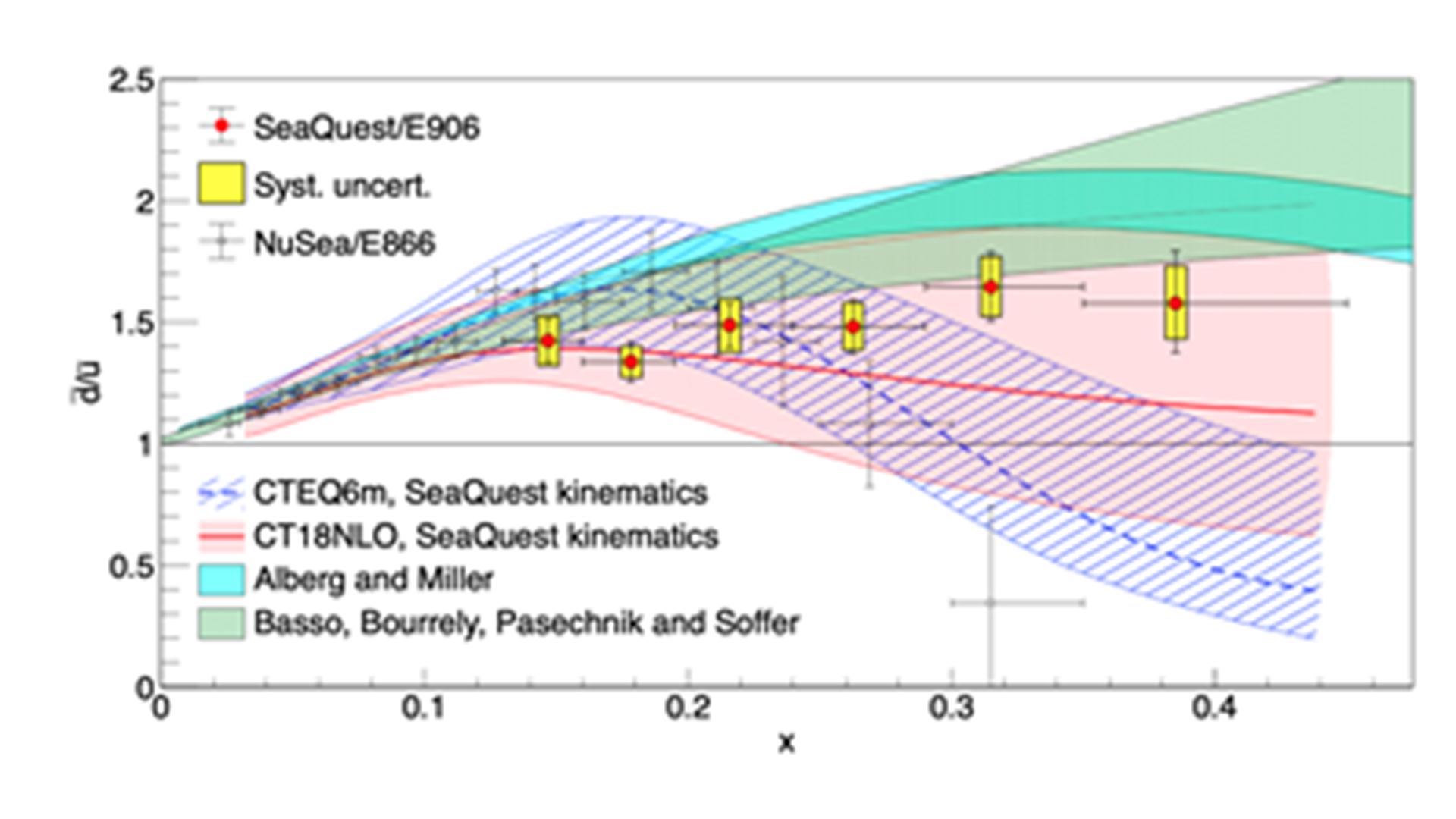
The best X data points from the E866 contain large statistical uncertainties, which requires new measurements with improved accuracy for large X regions. A SeaQuest (Fermilab E906) experiment using a new spectrometer and a 120 GEV proton beam recently reported its first results. ² Figure 2 shows the results of SeaQuest along with the E866. The SeaQuest results are qualitative agreement with that of the E866, but have a tension of the d̅(x)/u̅(x) ratio at the largest X remnants. SeaQuest results show that the d̅(x)/u̅(x) ratio for the entire measured X range is greater than unity.
Meaning and outlook
Some models, including the Meson Cloud Model, Chiral-Quark Model, Statistical Model, Chiral-Quark Soliton Model, and Instanton Model, increase in D̅(x)/u̅(x) as X increases in the small X region can be explained. (See References 3 and 4 for reviews). In most of these models, meson clouds are responsible for the flavor asymmetry between u̅(x) and d̅(x).
The importance of meson clouds to understand factors of nucleon forms on the relatively low Q² scale is well established, but meson clouds are able to produce a partially constructed flavor probed at a much higher Q² scale. It is interesting that it can also lead to asymmetry. d̅(x)/u̅(x) asymmetry is also utilized to extract the intrinsic photoquark sea content of protons. The asymmetry of the flavor of the four-light quark sea has also been investigated using lattice QCD technology.
Several recent global analyses include new SeaQuest results in addition to Star Collaboration’s recent W-boson production data. These new proton PDFs show that SeaQuest data significantly reduces the uncertainty of d̅(x)/u̅(x) at large x. SeaQuest data imposes new and harsh constraints on future efforts to extract proton parton distributions.
The unexpected discovery of the asymmetry of the flavor of the proton sea generated much interest and provided new insights into the origins of the nucleon sea. In large hadron criders (LHCs) or future electron ion corridors (EICs), the role of sea quarks becomes more important, as sea quark density is expected to rise at large Q², especially due to heavy taste. It will become. Small X-regions explored in these high-energy corridors. This provides an opportunity to study the flavour structure of the new motor sphere light Quark Sea and the lesser heavier quark Sea (S, C, B).
reference
“Improved measurement of d̅/u̅ asymmetry in the nuclear ocean,” Rs Towell et al. , (E866/Nusea Coll.), Phys. Rev. D65 (2001) 052002. “Asymmetry of antimatter in protons,” J. Doveet al (Seaquest Coll.), Nature 590 (2021) 561. and J.C. Peng, Prog. part. Nucl. Phys. 47 (2001) 203. “Flavor Structure of the Nucleon Sea,” WC Chang and JC Peng, Prog. part. Nucl. Phys. 79 (2014) 95.
This article will also be featured in the 20th edition of Quarterly Publishing.
Source link