Professor Peter Braun-Munzinger explores the work of GSI Helmholtzzentrum, highlighting its crucial role in the ALICE collaboration at CERN, current research directions, and key findings that may enhance our understanding of particle physics.
The GSI Helmholtzzentrum plays a pivotal role in advancing our understanding of particle physics through its contributions to the ALICE collaboration at CERN. With a dedicated team of researchers and a strong focus on the development and calibration of sophisticated detectors like the Time Projection Chamber (TPC) and the Transition Radiation Detector (TRD), GSI is at the forefront of groundbreaking experiments that probe the fundamental characteristics of the quark-gluon plasma (QGP).
This unique state of matter, which existed just moments after the Big Bang, offers insights into the behaviour of strong interactions and the early Universe. GSI not only participates in the operation of these vital detection systems but also drives significant scientific discoveries that enhance our understanding of the Universe.
To find out more, Professor Peter Braun-Munzinger delves into the work of GSI, highlighting their contributions and key findings that have emerged from their particle physics research efforts.
Can you explain the role of GSI in the ALICE collaboration? How does GSI support the operation and calibration of the Time Projection Chamber (TPC) and Transition Radiation Detector (TRD)?
As TPC Project leader from 1998 – 2010, I was responsible for the design, construction, and commissioning/calibration of the ALICE TPC. The TPC project comprised groups from major laboratories (CERN, GSI) and more than ten groups from different universities in Europe, the USA, and Asia. Particularly strong contributions came from the University of Frankfurt under the direction of Professor Harald Appelshaeuser and the University of Heidelberg under the direction of Professor Johanna Stachel.
The Alice Group at GSI currently has about 25 members, including master and doctoral students, postdocs, scientific staff, and two professors. Professor Silvia Masciocchi took over from me in 2011 and has led the group since I retired from the Technical University Darmstadt and took over the scientific direction of EMMI.
Just about everybody in the group participates in running shifts for the nine months of the year, during which the ALICE collaboration takes data. The calibration and operation of large detectors such as the TPC and the TRD is an extremely important but also demanding task that needs attention throughout the year to give constant feedback to the operation crews of the whole experiment and to provide information on data quality, potential problems, as well as results from on-line analysis.
The GSI group also played a very important role in the upgrade project for the TPC, which was prepared in the years 2013-2018, with the installation of new components in 2019-2021. Since 2022, the upgraded TPC has been reinstalled in the underground cavern of the experiment at CERN, and data has been taken at a ten-fold increased rate compared to what could be achieved in 2018. About half of 2022 was used for the complex tasks of calibration of the TPC. Since the Fall of 2022, the detector has been completely operational and participates in the ALICE data taking.
Operation and calibration of the ALICE TRD are mostly performed by the ALICE groups at Muenster and Heidelberg University. For detectors such as TPC and TRD, analysis of the data from the LHC collider requires continuous development of the analysis software, which currently also uses methods from machine learning and artificial intelligence. Many of the group members at all levels are involved.
What are some of the key findings or breakthroughs that GSI has contributed to through its work with ALICE?
The ALICE experiment is managed by a large collaboration where many tasks are shared by many ALICE groups from all over the world. So, it is not possible to single out particular groups or individuals. As one can imagine, the contributions by different groups of individuals range from new ideas for data analysis to developing new hardware pieces, from ideas for improving detector resolutions to developing new areas of focus for the whole experiment. This said, let me summarise key findings and breakthroughs where the GSI group and EMMI were particularly strongly involved.
The ALICE of the first ten years of measurements has shown that heavy-ion collisions at the LHC create conditions that very much exceed those needed to form the quark-gluon plasma QGP. The initial QGP temperature implied by our results is up to five times higher than the predicted QCD deconfinement temperature Tpc = 155–158 MeV and of the order of a trillion Kelvin, ~ 10⁵ times hotter than at the centre of the Sun.
The measurements of particle production yields integrated over all momenta have provided detailed information on the transition temperature to the QGP and demonstrated that this transition temperature coincides closely with the predicted deconfinement temperature Tpc = 155–158 MeV at LHC energies.
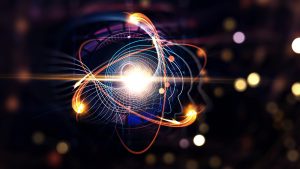
Significant modifications of the quarkonium binding in the QGP have been observed, with our results at the LHC showing new and striking features. For the J/ψ charmonium state, the QGP-induced suppression was shown to be counterbalanced by a strong regeneration effect, taking place during and/or at the fireball chemical freeze-out, that dominates charmonium production at low transverse momenta. This mechanism constitutes proof of deconfinement of the QGP, as it implies that coloured partons can move freely over distances much larger than the hadronic scale.
What is the significance of the ALICE tier-2 computing centre at GSI, and how does it benefit the ALICE collaboration?
The ALICE tier-2 centre at GSI is operated for 365 days a year and provides very important support for the complex task of providing the computing hardware and integrating the software developed elsewhere in the experiment so that the more than 50 Petabytes of ALICE data taken up to now can be efficiently analysed by ALICE users distributed all over the world. It also provides a hardware and software framework where new ideas and analysis tasks can be efficiently developed and quickly tested for further use in the overall analysis tasks.
The ALICE experiment is currently developing ALICE 3. What are the primary scientific objectives of the ALICE 3 experiment?
The main particle physics topics to be addressed with the new detector deal with the following fundamental questions:
How are colourless hadrons formed from the coloured and not freely observable quarks and gluons inside the QGP?
To address this question, a programme is envisioned to measure hadrons containing two or even three heavy quarks (charm and beauty) and study how they are formed from the deconfined constituents of the QGP. These hadrons are very unstable and decay rapidly, so their detection needs the vertex part of the planned ALICE 3 tracker; see question 5 below.
Such hadrons can only be formed from quarks that were produced in separate hard-scattering processes. If full thermal equilibrium is reached in Pb–Pb collisions, the production rates of such states are expected to be enhanced by up to three orders of magnitude with respect to pp collisions. This implies enormous sensitivity to the probability of combining independently produced quarks during hadronisation and to the degree of thermalisation.
To investigate the up-to-now poorly understood question of the restoration of chiral symmetry in the QGP, we plan to use the ALICE 3 detector to make high-rate, high-precision measurements of dilepton pairs emitted from the hot QGP.
A ‘forward conversion tracker’ also made from the same Si sensors will allow the measurement of ultra-soft photons in the forward direction. The measurements are designed to test directly the infrared limit of quantum field theories such as QED and QCD.
How does ALICE 3 improve upon the capabilities of the current ALICE detector? What new detector technologies and innovations are being introduced?
The planned ALICE 3 detector is a compact, large-acceptance tracking and particle-identification detector with excellent pointing resolution and high readout rates built around a completely new high-resolution tracking detector based on next-generation CMOS Si pixel sensors. These sensors were developed by members of the ALICE collaboration. The sensors are developed using 65 nm technology with high radiation tolerance and improved spatial resolution. The overall detector is ultra-thin: the foreseen material budget for the tracker is only 1% of the radiation length per layer for the outer tracker and only 0.05% per layer for the vertex tracker. The vertex part of the tracker is located inside the LHC accelerator beam pipe. The whole detector will be housed in a superconducting solenoidal magnet and complemented by special detectors for particle identification.
GSI has been heavily involved in the field of high-energy nuclear phenomenology. Can you detail your key areas of research in this field?
A small group of physicists (Anton Andronic from Muenster University, Peter Braun-Munzinger and Anar Rustamov from GSI, Krzysztof Redlich from Wroclaw University, and Johanna Stachel from the University of Heidelberg) are engaged in developing, together with doctoral students and postdocs, a programme to understand the phenomenology of particle production in collisions of high energy hadrons and nuclei.
The main idea behind this approach is contained in the following:
In relativistic nuclear collisions, the production of hadrons with light (u,d,s) quarks is quantitatively described in the framework of the Statistical Hadronization Model (SHM). Charm quarks are dominantly produced in initial hard collisions but interact strongly in the hot fireball and thermalise. Therefore, charmed hadrons can be incorporated into the SHM by treating charm quarks as ’impurities’ with thermal distributions, while the total charm content of the fireball is fixed by the measured open charm cross-section. We call this model SHMc and demonstrate that with SHMc, the measured multiplicities of single charm hadrons in lead-lead collisions at LHC energies can be well described with the same thermal parameters as for (u,d,s) hadrons.
What are some of the recent breakthroughs or discoveries made by the phenomenology group in the field of high-energy nuclear phenomenology?
Some of the SHMc predictions have already been verified, particularly in the charmonium sector. Charmonia are particular hadrons made from a charm quark and its anti-particle. The successful prediction of charmonium yields is demonstrated in two important recent publications that have appeared in Nature 561 (2018) 7723, 321-330 and in Journal of High Energy Physics 07 (2021) 035.
The SHMc model is currently extended to include doubly- and triply-charmed hadrons. First predictions for production probabilities of such exotic states exhibit a characteristic and quite spectacular enhancement hierarchy. Further measurements on this subject will be undertaken in the coming six years of ALICE operation. Complete tests are planned within the new ALICE 3 experiment.
How does GSI’s research on high-energy nuclear collisions contribute to broader scientific goals, such as understanding the early Universe or the behaviour of matter under extreme conditions?
Note that the matter produced in high energy Pb-Pb collisions is, in a number of aspects, very similar to that which existed in the early Universe in the first microseconds after the Big Bang. In particular, this matter contains equal portions of matter and anti-matter and undergoes a similar phase transition between hadronic matter and quark-gluon plasma.
Whether this phase transition observed at the ALICE collaboration at the CERN LHC collider can ever be detected by astronomical observations in the early Universe is highly uncertain. The ALICE measurements at the CERN LHC are consequently also of high relevance for our understanding of the structure of matter in the early Universe.
GSI is also home to the ExTreMe Matter Institute (EMMI). What are the primary research areas of EMMI, and how do they contribute to our understanding of matter under extreme conditions?
EMMI was funded in 2008 in the framework of the Helmholtz Alliance 2008-2015 entitled ‘Cosmic Matter in the Laboratory.’ Since 2016, EMMI has been operating as a regular department of GSI. It currently has 12 partner institutions in Germany, France, the USA, and Japan. The current scientific director is Peter Braun-Munzinger, and Carlo Ewerz (GSI) is the scientific coordinator.
Its main research areas (among other related topics) are:
Quark-gluon plasma, the phase diagram of strongly interacting matter, and hadron physics
Structure and dynamics of neutron-rich systems from the laboratory to the stars
Plasma physics
Atomic physics and ultracold gases
The focus is on delineating common concepts between these very different research areas requiring a combination of different theoretical methods, such as:
BEC and BCS
QGP and ultracold Fermi gases
Holographic duality relating QCD to black holes
Multi-messenger astronomy: neutron star mergers kilonovas
Hydrodynamics, turbulence
Hence, a central goal of EMMI is to act as a think tank and provide an intellectual environment for extreme matter research (at GSI and beyond) aiming at:
Interdisciplinary scientific events of the highest quality
Strong promotion of early-career researchers
Network among two Helmholtz centres and 11 top national and international laboratories and universities
Please note, this article will also appear in the 21st edition of our quarterly publication.
Source link