By advancing the production and application of isotopes like actinium-225, the research and development teams at TRIUMF have the potential to revolutionise cancer care.
Radiopharmaceuticals are specialised compounds that combine a radioactive isotope with a pharmaceutical molecule to target specific cells or tissues within the body. These compounds play a critical role in both diagnostic imaging and therapeutic treatments, with particularly impactful benefits within the field of nuclear medicine.
Historically, researchers and doctors have leveraged the unique characteristics of isotopes and radioactive decay – the process by which the atomic nucleus spontaneously gives off a parcel of energy in the form of a particle – to visualise how diseases have established or are progressing. This area of development has provided some of the most powerful tools in the modern medical toolbox, including the PET and SPECT scanning techniques used to assess heart and bone diseases, cancer, and other diseases.
Now, novel research and advances in radiopharmaceutical development are enabling us to use isotopes not just for scanning and diagnosing disease but for treating diseases directly. One class of isotopes in particular, alpha-emitting isotopes, holds tremendous promise to revolutionise the way we treat cancer and other diseases and significantly improve health outcomes. The only problem? Historically, we just haven’t had enough production capacity to make enough of the isotopes. Some small amounts can be developed from non-renewable sources like spent nuclear fuel rods, but not enough to enable large-scale clinical trials that are required for health authority approvals.
At TRIUMF, Canada’s particle accelerator centre, research and engineering teams are developing novel techniques to produce these isotopes using compact but powerful accelerators called cyclotrons. Using a suite of onsite cyclotrons, researchers are exploring new ways to create significant quantities of isotopes that are critical to advancing the burgeoning field of radiopharmaceuticals.
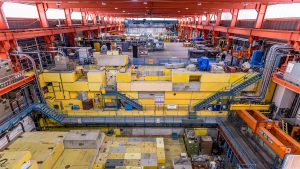
One isotope that has emerged at the forefront of this new paradigm is actinium-225 – dubbed the ‘world’s rarest drug’. However, to understand the promise of actinium-225 and the potential of alpha-emitting isotopes, we must first understand how isotopes are used in the body.
Leveraging fundamental physics research to visualise and treat cancer and other diseases
The field of nuclear medicine, which sees researchers and medical professionals leveraging radioactive particles as medical tools, is rooted in decades of fundamental physics research. Intense focus on the field of subatomic physics in the middle of the 20th century brought a wide variety of innovations and advances that stemmed from our newfound understanding of the atomic nucleus.
Building on our knowledge of how atoms bind together and how they split apart or decay, researchers began delving into the many applications and real-world uses for radioactive particles. By the early 1970s, most organs within the body were able to be visualised using nuclear medicine techniques – the foremost among them being positron emission tomography (PET) scanning.
In PET and other imaging techniques like Single Photon Emission Computed Tomography (SPECT), a patient is administered a small dose of a diagnostic radiopharmaceutical – a solution that contains both an endogenous human molecule (for instance, a protein or hormone) that is chemically bound to a radioactive isotope. This bound molecule will, based on the nature of the endogenous molecule, travel to the destined part of the body; for instance, a hormone would travel to the part of the body where the hormone is typically expressed or processed, whereas a bound sugar molecule would travel to any major muscle or system where sugar is typically used. As the molecule accumulates in its destination within the body, the ‘diagnostic tool’ section of the molecule – the radioactive isotope – decays, a naturally-occurring process wherein the atom emits energy in the form of gamma rays or positrons, which can then be detected by imaging devices, not unlike how a typical camera detects a photon. With enough of these decay events and emitted radioactive particles, the imaging device can begin to triangulate and visualise an ‘image’ of the internal human body: a diagnostic scan. These nuclear medicine diagnostic scans have become critical tools for doctors, who can in turn visualise internal organs, detect abnormalities, and monitor various physiological processes.
By contrast, therapeutic radiopharmaceuticals are used for targeted treatment of diseases, especially cancer.
Using radioactive isotopes that emit alpha or beta radiation (instead of gamma rays or photons), these radiopharmaceuticals are guided to a particular area of the body where they decay and deliver a minute but impactful dose of radiation that acts across mere millimetres. This ‘nuclear payload’ is enough to kill cells within the immediate vicinity (for instance, within or near a tumour) but small and targeted enough that it does not impact other cells or systems beyond the localised area of action. Further, the molecules can be engineered to produce relatively safe ‘daughter’ decay products, and to be excreted within a short period of time, meaning there is no persistent risk for the patient or the downstream effects to the environment. Examples of therapeutic radiopharmaceuticals include iodine-131, used to treat thyroid cancer, and lutetium-177, which is increasingly used for certain types of neuroendocrine and other tumours – and actinium-225, an alpha-emitting isotope that shows tremendous potential to treat metastatic prostate cancer.
Actinium – the world’s (rarest) wonder drug
Actinium-225 is a rare and highly radioactive isotope that has emerged as one of the most promising candidates for targeted alpha-particle therapy, with significant promise in the treatment of cancer and scenarios where conventional therapies have limited effectiveness or cause undesirable side effects. Actinium-225 decays into a series of isotopes; during this decay process, it emits high-energy alpha particles. These alpha particles have a very short range (approximately 50 to 100 microns), meaning they can cause significant damage to the targeted cells without affecting surrounding tissues. This characteristic makes actinium-225 ideal for attacking solid tumours and cancerous cells that are difficult to treat with conventional radiation or chemotherapy. This can result in fewer side effects compared to traditional radiation therapy, where healthy tissue can often be damaged by the radiation.
As research continues and production capabilities improve, actinium-225 is poised to become a game-changer in the field of targeted cancer therapy, offering a highly effective and minimally invasive treatment option for patients with otherwise difficult-to-treat cancers. However, while actinium-225 holds immense promise, there are still several challenges to overcome in its clinical application; for instance, further research is needed to establish optimal dosages, treatment regimens, and safety protocols. The primary limitation to advancing this promising therapy, though, is that there is simply not enough of it to study. The production of actinium-225 is currently extremely limited, and non-trivial to pursue.
The burgeoning role of particle accelerators in producing alpha-emitting isotopes
Particle accelerators are machines that accelerate charged particles to high velocities and then direct them to collide with target materials. The interaction of the accelerated particles with the target material can produce a variety of nuclear reactions, leading to the production of different isotopes.
While particle accelerators have been used for many years in nuclear physics research and medical applications, their role in the production of therapeutic isotopes has become increasingly important in recent years. As demand for alpha-emitting isotopes like actinium-225 grows, the need for reliable and efficient production methods has also increased. Particle accelerators offer several advantages in this regard. First, they allow for the precise control of the nuclear reactions used to produce isotopes, ensuring high yields and purity. Second, accelerators can be used to produce isotopes consistently and reliably, making them ideal for producing rare isotopes like actinium-225, which are not readily available from other sources. This would allow local or regional health centres to produce a daily run of isotopes for a cohort of patients; provide the treatment; and then repeat the process.
Beyond actinium-225, particle accelerators are used to produce a wide range of other therapeutic and diagnostic isotopes, such as iodine-131 and the most commonly used medical isotope, technetium-99m. As the demand for radiopharmaceuticals for research and directly treating patients continues to grow, so too will the need for advanced accelerator technology to meet this demand.
TRIUMF is working to address this need.
TRIUMF’s role in producing actinium-225
Founded in 1968, TRIUMF is a major Canadian research facility located in Vancouver, BC. The laboratory is home to a diverse and globally unique community of world-leading research teams investigating fundamental questions in particle and nuclear physics; materials sciences; nuclear medicine; and more.
TRIUMF also plays a unique and critical role in the production of actinium-225. TRIUMF’s life sciences research teams collaborate with world-leading accelerator experts to not only produce significant quantities of actinium-225 using the on-site (and world’s largest!) 520 MeV cyclotron, but also drive advances in the techniques and methodologies in the production paradigm, increasing efficiency and streamlining workflows. With world-leading accelerator infrastructure and a globally unique and diverse roster of researchers, engineers, and technicians, TRIUMF anticipates becoming a major leader in global cyclotron-based production of actinium-225, positioning itself as a key player in the emerging field of targeted alpha-particle therapy.
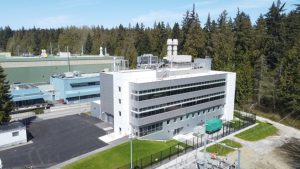
In addition, TRIUMF continues to grow its capacity for research and production of critically-needed medical isotopes. Now in its final stages, the Institute for Advanced Medical Isotopes (IAMI) will serve as TRIUMF’s flagship nuclear medicine research facility, enabling additional research capacity for actinium-225 and other alpha-emitting isotopes, as well as production capacity for routine radiopharmaceuticals.
As demand for alpha-emitting isotopes increases, teams at TRIUMF and IAMI will be working to scale up production while maintaining the high quality of the various radiopharmaceuticals. The laboratory collaborates with various national and international medical institutions, universities, and pharmaceutical companies to advance the development and clinical application of actinium-225-based treatments.
TRIUMF’s contribution to the field of radiopharmaceuticals is further amplified by its role in advancing particle accelerator technology. Leveraging over 50 years of expertise in the areas of accelerators and target systems, as well as world-unique infrastructure on its 13.5-acre campus, TRIUMF continues to push the envelope and explore new methods for improving the efficiency and scalability of isotope production. As Canada’s premiere accelerator research centre, TRIUMF will be critical in meeting the increasing national and global demand for radiopharmaceuticals in the coming years. By advancing the production and application of isotopes like actinium-225, TRIUMF is paving the way for new and innovative treatments that have the potential to revolutionise cancer care.
A bright future ahead
Radiopharmaceuticals, particularly those that utilise alpha-emitting isotopes like actinium-225, are at the cutting edge of cancer treatment and diagnosis. The promise of these therapies lies in their ability to target specific tumour cells while minimising damage to surrounding healthy tissue. Actinium-225 shows great potential for treating various cancers and solid tumours that have proven resistant to traditional therapies. Further, the role of particle accelerators in producing these isotopes cannot be overstated. Facilities like TRIUMF are at the forefront of this development, providing the infrastructure and expertise necessary to meet the growing demand for radiopharmaceuticals. As advancements in accelerator technology continue, the availability of rare and highly effective isotopes will increase, making targeted alpha-particle therapy a more accessible and effective treatment option for cancer patients worldwide.
The future of radiopharmaceuticals is bright and, with continued research and innovation, these therapies may one day become a standard of care for many cancer patients, offering hope for better outcomes and improved quality of life.
Please note, this article will also appear in the 21st edition of our quarterly publication.
Source link