The Canadian Light Source is being used to help combat various real-world problems in a wide range of settings, from vaccine research to food production.
Scientists from around the world are using the Canadian Light Source (CLS) at the University of Saskatchewan (USask) to solve some of the toughest challenges in health, agriculture, the environment, and advanced materials.
The CLS is the only Canadian synchrotron and one of only a couple of dozen similar research facilities around the world. In simple terms, a synchrotron produces ultrabright light that enables researchers to study structural and chemical properties of materials at the molecular level.
From helping in the fight against emerging diseases and creating new cancer-fighting drugs, to developing more nutritious and climate-resistant crops and supporting clean-tech and green mining processes, CLS’s infrastructure and experts help researchers solve problems, train the next generation of scientists, and support industries to become more efficient and sustainable.
This article will discuss several examples of discoveries enabled by beamlines at the CLS during 2024.
Health: Newly discovered protein stops DNA damage
Using the CLS, researchers from Western University have discovered a protein that has the never-before-seen ability to stop DNA damage in its tracks. The finding could provide the foundation for developing everything from vaccines against cancer, to crops that can withstand the increasingly harsh growing conditions brought on by climate change.
The researchers found the protein – called DdrC (for DNA Damage Repair Protein C) – in a common bacterium called Deinococcus radiodurans (D. radiodurans), which has the decidedly uncommon ability to survive conditions that damage DNA – for example, 5,000 to 10,000 times the radiation that would kill a regular human cell. Lead researcher Robert Szabla said Deinococcus also excels in repairing DNA that has already been damaged.
“It’s as if you had a player in the NFL who plays every game without a helmet or pads,” said Szabla, a grad student in Western’s Department of Biochemistry.
“He’d end up with a concussion and multiple broken bones every single game, but then miraculously make a full recovery overnight in time for practice the next day.”
Szabla and his colleagues discovered that DdrC is a key player in this repair process. Every cell has a DNA repair mechanism to fix damage.
Szabla continued: “With a human cell, if there are any more than two breaks in the entire billion base pair genome, it can’t fix itself and it dies. But in the case of DdrC, this unique protein helps the cell to repair hundreds of broken DNA fragments into a coherent genome.”
Szabla and his team used the CLS to determine the 3D shape of the protein, from which they then worked backwards to better understand its ‘superpower’ to neutralise DNA damage.
“The Canadian Light Source was instrumental in that,” said Szabla. “It’s the most powerful X-ray source in Canada.” The group’s findings were published in the journal Nucleic Acids Research.
The DdrC scans for breaks along the DNA and, when it detects one, it snaps shut – like a mousetrap. This trapping action has two key functions: it neutralises it (the DNA damage), and prevents the break from getting damaged further. And it acts like a little molecular beacon. It tells the cell ‘Hey, over here. There’s damage. Come fix it.’
Typically, said Szabla, proteins form complicated networks that enable them to carry out a function. DdrC appears to be something of an outlier, in that it performs its function all on its own, without the need for other proteins. The team was curious whether the protein might function as a ‘plug-in’ for other DNA repair systems. They tested this by adding it to a different bacterium: E-coli.
“To our huge surprise, it actually made the bacterium over 40 times more resistant to UV radiation damage,” he said. “This seems to be a rare example where you have one protein and it really is like a standalone machine.”
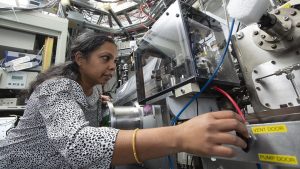
Szabla said that, in theory, this gene could be introduced into any organism – plants, animals, humans – and it should increase the DNA repair efficiency of that organism’s cells.
“The ability to rearrange and edit and manipulate DNA in specific ways is the holy grail in biotechnology,” he said. “What if you had a scanning system such as DdrC which patrolled your cells and neutralised damage when it happened? This might form the basis of a potential cancer vaccine.”
The Western team is just getting started studying Deinococcus.
“DdrC is just one out of hundreds of potentially useful proteins in this bacterium. The next step is to prod further, look at what else this cell uses to fix its own genome – because we’re sure to find many more tools where we have no idea how they work or how they’re going to be useful until we look.”
Agriculture and food production: Finetuning fertilisers to boost crop yields
Worldwide, many agricultural soils are deficient in the nutrient zinc – even though farmers use fertilisers enriched with the element. This limits crop yields and reduces food quality. It’s estimated that roughly a third of the global population consumes foods low in zinc, which can increase sickness and death in early childhood, as well as impaired growth and cognition.
Using the CLS, researchers from the University of Adelaide in Australia investigated how to manufacture more efficient zinc-enriched fertilisers. The HXMA beamline at CLS enabled the researchers to examine at the molecular level what happens to the water solubility of zinc (its ability to dissolve in water) when it’s added to ammonium phosphate fertiliser.
Rodrigo da Silva, from the University of Adelaide, said: “Going into the project, our group thought the type of zinc compound would be a good predictor of a fertiliser’s solubility.
“However, the CLS beamline enabled us to understand that the agronomic performance cannot be predicted based on what form of zinc is present in the fertiliser granules. Instead, the pH drives the fertiliser zinc solubility and availability to the crops.”
Dr da Silva and colleagues found that, when zinc is added to phosphate fertiliser, it forms a range of different zinc phosphate compounds. However, its solubility was not related to the relative abundance of these compounds, but to fertiliser pH. This means that zinc added to more alkaline phosphate fertilisers, such as diammonium phosphate, will have very low solubility and hence low agronomic effectiveness for crop uptake.
The University of Adelaide team found that lowering fertiliser pH by spraying the granules with an acid solution further increases the solubility and availability of zinc compared to current commercial products. The researchers also developed an additional method to increase Zn water solubility by putting a barrier coating on fertiliser granules, to separate the zinc from the phosphate. They showed that there was more uptake of zinc by plants treated with the barrier-coating fertiliser.
The Mosaic Company, which funded this research, has already patented both of these new technologies in conjunction with the University of Adelaide’s Fertiliser Technology Research Centre. Mosaic is the world’s leading producer of concentrated phosphate and potash. The findings are published in the Soil Science Society of America Journal.
“It is crucial to improve zinc fertilisation practices, to maximise yields and produce more nutritional food,” said da Silva. “This research can help the industry produce more efficient fertilisers.”
Energy and advanced materials: New type of battery could outlast EVs and still be used for grid energy storage
There’s a big push underway to increase the lifespan of lithium-ion batteries powering electric vehicles (EVs) on the road today. By law, in the US, these cells must be able to hold 80% of their original full charge after eight years of operation.
However, many industry experts believe we need batteries that last decades – so that once they’re no longer robust enough for use in EVs, we can put them to use in ‘second-life applications’ – such as bundling them together to store wind and solar energy to power the electrical grid.
Researchers from Dalhousie University used the CLS to analyse a new type of lithium-ion battery material – called a single-crystal electrode – that’s been charging and discharging non-stop in a Halifax lab for more than six years. It lasted more than 20,000 cycles before it hit the 80% capacity cutoff. That translates to driving a jaw-dropping eight million kilometres. As part of the study, the researchers compared the new type of battery – which has only recently come to market – to a regular lithium-ion battery that lasted 2,400 cycles before it reached the 80% cutoff.
“The main focus of our research was to understand how damage and fatigue inside a battery progresses over time, and how we can prevent it,” said Toby Bond, a senior scientist at the CLS, who conducted the research for his PhD, under the supervision of Professor Jeff Dahn, Professor Emeritus and Principal Investigator (NSERC/Tesla Canada/Dalhousie Alliance Grant) at Dalhousie University. The study was funded by Tesla Canada and NSERC under the Alliance Grant program.
Things got very interesting, said Bond, when the scientists used the ultrabright synchrotron light to peer inside the two batteries. When they looked at the inner workings of the regular lithium-ion battery, they saw an extensive amount of microscopic cracking in the electrode material, caused by repeated charging and discharging. The lithium, he explains, forces the atoms in the battery material apart and causes expansion and contraction of the material.
“Eventually, there were so many cracks that the electrode was essentially pulverised.”
However, when the researchers looked at the single crystal electrode battery, they saw next to no evidence of this mechanical stress. “In our images, it looked very much like a brand-new cell. We could almost not tell the difference.”
Bond attributes the near absence of degradation in the new style battery to the difference in the shape and behaviour of the particles that make up the battery electrodes. In the regular battery, the battery electrodes are made up of tiny particles up to 50 times smaller than the width of a hair. If you zoom in on these particles, they are composed of even tinier crystals that are bunched together like snowflakes in a snowball. The single crystal is, as its name implies, one big crystal: it’s more like an ice cube. “If you have a snowball in one hand, and an ice cube in the other, it’s a lot easier to crush the snowball,” said Bond. “The ice cube is much more resistant to mechanical stress and strain.”
While researchers have for some time known that this new battery type resists the micro cracking that lithium-ion batteries are so susceptible to, this is the first time anyone has studied a cell that’s been cycled for so long.
Bond added: “The great thing about doing this kind of measurement at a synchrotron is we can actually look at this at a microscopic level without having to take the cell apart. Once we cycle a cell for six years, you really don’t want to take it apart – it’s very precious, it’s very valuable to us, with so much information contained within it.”
Bond said what’s most exciting about the research is that it suggests we may be near the point where the battery is no longer the limiting component in an EV – as it may outlast the other parts of the car. “We really need these vehicles to last as long as possible, because the longer you drive them, the better its improvement on the carbon footprint is,” said Bond. As well, if battery packs can outlast the vehicle, you can use them for mass energy storage – where the energy density that’s critical for powering an EV – doesn’t matter as much.
The new batteries are already being produced commercially, said Bond, and their use should ramp up significantly within the next couple of years. “I think work like this just helps underscore how reliable they are, and it should help companies that are manufacturing and using these batteries to plan for the long term.”
Environment: Researchers develop reusable ‘sponge’ for soaking up marine oil spills – even in chilly northern waters
Oil spills, if not cleaned up quickly and effectively, can cause lasting damage to marine and coastal environments. That’s why a team of North American researchers are developing a new sponge-like material that is not only effective at grabbing and holding oil on its surface (adsorption) but can also be reused again and again – even in icy Canadian waters.
The special material – called CNF-SP aerogel – combines a biodegradable cellulose-based material with a substance called spiropyran, a light-sensitive material. Spiropyran has a unique ‘switchable’ property that allows the aerogel to go between being oil-sorbent and oil-repellent, just like a kitchen sponge that can be used to soak up and squeeze out water.
“Once spiropyran has been added to the aerogel, after each usage we just switch the light condition,” explained Dr Baiyu Helen Zhang, Professor and Canada Research Chair at Memorial University, Newfoundland. “We used the aerogel as an oil sorbent under visible light. After oil adsorption, we switched the light condition to UV light. This switch helped the sponge to release the oil.”
The material continues soaking up and releasing oil, even when the water temperature drops, according to Dr Xiujuan Chen, Assistant Professor at University of Texas – Arlington.
“We found that, when we tested the oil sorbent’s performance under different kinds of environmental conditions, it had a very good performance in a cold environment. This is quite useful for cold winter seasons, particularly for Canada,” said Dr Chen.
The researchers used the CLS’ Mid-IR beamline to examine the characteristics of the aerogel before and after exposing it to visible and UV light. From here, the researchers are looking to scale up their research with large pilot studies and testing the material in the field.
“The CLS has very unique infrastructure that supports students and researchers like us to conduct many kinds of very exciting research and to contribute to scientific knowledge and engineering applications,” said Zhang.
Since starting operations in 2005, the CLS has enabled more than 5,700 users to publish close to 8,000 scientific publications — including over 4,600 peer-reviewed articles. The facility has played host to 1,100 collaborations involving 45 different countries.
Please note, this article will also appear in the 21st edition of our quarterly publication.
Source link