Rebecca Tadesse and Gabriele Grassi of the Radioactive Waste Management and Decommissioning Department of the OECD Nuclear Energy Agency discuss the importance of international cooperation for effective radioactive waste management.
Increasing countries are considering building nuclear facilities to ensure energy supply and reduce carbon emissions. As a result, the effective management of radioactive waste generated by these facilities has attracted new attention.
The number of countries expressing interest in nuclear energy programs increased in the 2020s, with 30 countries declaring three times the nuclear energy capacity in 2023 by 2050 as part of their zero commitment. Meanwhile, the development of more diverse new and advanced nuclear technologies is accelerating. This advancement involves scientists, regulators, disposal facility developers and operators, policymakers and other experts in the life cycle of potentially new forms of spent nuclear fuel (SNF) and radioactive waste. You need to reevaluate how you manage it.
Since the start of private nuclear energy over 70 years ago, the sector has been responsible for the entire life cycle of its materials. Due to the global nature of the industry, there is the urgency to develop effective low-carbon energy solutions, and the speed of innovation, management of radioactive waste management is most effectively pursued through international collaboration. Masu. Such collaborations include sharing information, harmonizing approaches, and avoiding duplication of work. The Nuclear Energy Agency (NEA) has been active in research, technology and policy on radioactive waste management since 1958, and is committed to supporting 34 member countries with this long-term effort. .
Spent nuclear fuel and high levels of radioactive waste
Radioactive materials produced in nuclear reactors are usually classified within each national framework based on radioactivity and “damping” time. From very low levels of waste to low levels of waste, medium levels of waste, high levels of waste (HLW). Much of the radioactivity in waste will disappear or fade over centuries, but a very small proportion will remain dangerous for thousands of years.
The SNF is stored in temporary storage facilities (wet pools and dry casks) awaiting final disposal. A small amount of HLW remains after reprocessing, and requires long-term management. The extended decay times of SNF/HLW have sparked debate in some parts of the world about the long-term sustainability of nuclear energy. However, the global scientific consensus is clear. Permanent disposal of SNF/HLW in deep geological repository (DGR) is a safe and effective solution. By separating radioactive waste from the biosphere for thousands of years, DGR offers a reliable way to ensure environmental and human safety over the long term.
Deep geological abandonment
The DGR is an engineered tunnel excavated several hundred meters underground with carefully selected host rock formations. These repositories house SNF/HLW in a secure canister. Decades of research around the world have made it possible for independent national regulators to support DGR as the optimal solution for permanent disposal of SNF/HLW, based on internationally accepted standards of radiation protection. It has become.
DGRs are constructed within host rocks such as clay, granite, or salt layers, providing a stable geological environment and “passive safety” for waste disposal. This ensures that human intervention will be required for centuries to ensure that waste is properly buried and not disturbed. Extensive geological studies have examined their formation and understand their past behavior over hundreds of thousands, and even millions of years.
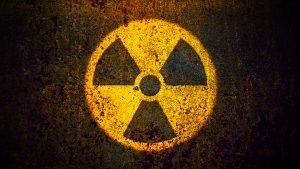
In industrial terminology, the amount of SNF/HLW is relatively small. For example, the United States, which has the largest number of nuclear reactors in the world, has produced a cumulative total of approximately 90,000 meters of spent fuel since the 1950s. This amount could fit in a soccer field below 10m deep.
Efforts to establish DGRS have progressed in various countries, with many underground labs (URLs) being constructed to conduct experiments on rock formations similar to those targeted at DGR. The study focuses on topics such as radionuclide and gas migration in rock formations, self-repair behavior of clay host rocks, thermal testing, fracture detection methods, and changes in geochemical conditions near DGR tunnels. These studies also tested the techniques used during DGR operations and final closure.
Finland has so far been the most advanced country in its DGR programme, and Olkiluoto Island’s Onkalo site is expected to be in operation in the coming years. is. The facility currently consists of a 10km tunnel and a depth of approximately 450m. Once the repository was fully operational, an additional 40km was planned for the next century.
International experiences such as Finland emphasize that high quality stakeholder engagement and well-designed public policy are key to successful DGR programs. Countries such as Canada, France, Sweden, and Switzerland are also advancing their DGR programmes by identifying appropriate sites and establishing necessary regulatory and legal frameworks with effective stakeholder involvement.
It is important to ensure public trust and obtain a “social license” to build and operate the repository. DGR safely separates radioactive waste for tens of thousands of years. However, this extended time frame poses unique challenges, such as how to convey the importance and purpose of the repository to future generations. Advances in science and technology could encourage future societies to enhance repository functions and change their operations.
From ideas to implementation
A broad and transparent dialogue with all stakeholders is essential when planning your DGR. Stakeholder engagement can take many forms, including expert consultations, committees, community meetings, public hearings, and legislative sessions. By encouraging open and continuous communication, countries can improve their technical choices while building social trust, thereby minimizing the risk of expensive policy U-turns over time. can. ¹
The decision to build a DGR requires careful planning and the creation of a national policy and regulatory framework. This includes planning the costs of projects that will live longer than any of the politicians, scientists or experts currently involved. Important considerations include determining who will bear the costs of radioactive waste management along the entire lifecycle, including the IF where the government asserts its costs and liability. Masu. “Safety Case” Update – A comprehensive demonstration of safety. These updates integrate new research results, advances in site characterization, and improved repository design. To support this, NEA maintains a database of features, events, and processes (FEPs) that can inform the scenarios to be investigated within a safety case.
Although DGRs are designed to provide passive safety, the possibility of careless human obstruction in the distant future, for example, through excavation of underground resources, remains a concern. resource. Furthermore, the safety case rigorously examines the resilience of the DGR to such scenarios. Also, efforts are underway to institutionalize the memory of DGR sites for future generations, both nationally and locally. A monument, museum, or community center serves as a permanent reminder of the importance of a repository, allowing knowledge to be safely transferred over centuries.
The uncertainty inherent to such long-term programs means flexibility is required throughout the decision-making process, including the possibility of decision-making reversal. This means, for example, ensuring the ability to acquire waste as already stipulated in the DGR. This will provide future generations with the ability to make uniquely informed decisions about the management of the site and SNF/HLW, taking into account technological advancements and changing political and social views.
New nuclear technology
The growing interest in nuclear energy as a low carbon source has led to the development of numerous new reactor concepts due to the growing interest as a low carbon source for electricity and heat. Unlike in the past, when public sector companies traditionally dominated the nuclear sector, the private sector, including venture capital-backed startups, has invested heavily in new technologies. These innovations include Generation IV Reactor Technologies and a Small Modular Reactor (SMR). The SMR is portable, factory-made and designed to have an output of less than 300 megawatts (MWE). Leading technology companies have expressed interest in supplying data centers to SMRS. Data center energy requirements have increased significantly for applications in demand, such as artificial intelligence. New nuclear technologies are also particularly relevant to difficult sectors that are mitigating, such as mining, transport, and a variety of industrial processes that require both power and heat, remote or off-grid locations.
Many of these emerging technologies use new materials and may require a variety of setups in terms of seating, licensing, supply chain, financing and fuel cycle management. This evolution has a major impact on radioactive waste management. As new reactor types generate SNF/HLW, it is important to address these challenges during the design stage.
To support this effort, NEA has developed a new international research project entitled Waste Integration for Small and Advanced Reactor Design (Wisard). , and discarded. ⁶ Addressing these questions during the early design stage of new nuclear technology can avoid costly design changes later in the development stage and build public trust in the sustainability of innovation. The project is expected to begin in early 2025 and will be added to the various initiatives and platforms provided by NEA, promoting information sharing between countries, sectors and industries, and understanding and management of radioactive waste management. Continuously promote final disposal. HLW.
reference
NEA (2020), Management and Disposal of High Levels of Radioactive Waste: Global Progress and Solutions, OECD Publishing, www.oecd-nea.org/jcms/pl_32567/management-and-disposal-of-high-radioactive -waste- global-progress-and-solutions (accessed January 7, 2025) doe (2020), 5 fast facts about spent nuclear fuel, www.energy.gov/ne/articles/5-fast-facts-abouts-spent- nucleut-fuel (accessed January 7, 2025) Posiva (2024), “Introduction to Onkalo and its operation principles”, Posiva’s website, www.posiva.fi/en/index/pressreleasestockexchangereleases/2024/thisisonkaloandthisisihowitworks.html (2023) NEA (2023). www.oecd-nea.org/jcms/pl_59705/ensuring-the-adequacy-funding-for-decommissioning-and-radioactive-waste-management (accessed January 7, 2024) NEA (2024): 2nd edition, OECD Publishing, Paris, www.oecd-nea.org/jcms/pl_73678/nea-small-modular-modular-modular-reactor-smr-dashboard (accessed January 7, 2025) NEA, (2024a), “Small and NEA Project on Waste Integration in Advanced Reactor Design (Wisard), https://www.oecd-nea.org/wisard (accessed January 7, 2025)
This article will also be featured in the 21st edition of Quarterly Publication.
Source link